The capture and use of carbon dioxide offers enormous potential to reduce environmental impacts and, when combined with other technologies, can play a crucial role in achieving emission reductions. Moreover, due to its unique properties, carbon dioxide is a versatile compound with many potential practical applications. This overview will explain why investing in this market can be beneficial now.
1. The necessity of CO2-utilization
Since the beginning of industrialization, an increasing amount of carbon dioxide (CO2) has been emitted into the atmosphere worldwide through the combustion of fossil fuels. As a result, natural carbon sinks such as oceans and forests with their plants, algae and soil microorganisms are no longer able to fully capture or convert the additional CO2 generated. Consequently, the saturation of the atmosphere with carbon dioxide has risen continuously and once again reached an all-time high in 2021.
1.1. Record value of 421 ppm in 2021
While the annual increase at the measuring station on Mauna Loa (Hawaii) was still 0.55 ppm carbon dioxide on average in the 1950s, the global trend has risen to 2.24 ppm per year in the past 15 years, 2.28 ppm per year at Mauna Loa. Compared to the 1950s, the global CO2 increase has nearly quadrupled.
Over the same period, the global CO2 concentration has risen from around 315 ppm (1958) to the current 418.56 ppm (June 2022), an increase of around 100 ppm, equivalent to an increase of 30%. On April 8, 2021, the CO2 concentration reached a record 421 ppm . 1
Figure 1: Increase in CO2 concentration to 421 ppm in 2021. Adapted from 2.
On a global average, the earth's surface temperature has risen by more than one degree - despite enormous efforts over the last ten years. The goal of the Paris climate agreement cannot be achieved solely by reducing CO2 emissions.
Figure 2: Atmospheric CO2-concentration on a monthly average in Germany, Hawaii and worldwide 3.
1.2. The consequence: Negative emissions of 10 Gt per year by 2050
The results of the IPCC Special Report "Global Warming of 1.5 °C" show that achieving net zero emissions by 2050 is not possible without large-scale use of technologies to remove CO2. In order to stabilize the climate, 10 Gt of CO2 must be removed from the atmosphere annually by 2050, while at the same time, no new emissions should arise. 4
Nevertheless, the challenges will not diminish since 730 Gt of CO2 should be removed from the atmosphere from 2050 until 2100. That is approximately 20 times the amount of CO2 emitted globally per year today.
Consequently, no sector will be spared from far-reaching emission reductions and new technologies will be required to meet these reductions. In addition to the energy and transport sectors, the focus is increasingly on CO2 emissions from industry, in particular the energy- and resource-intensive sectors.
Carbon Capture and Utilization (CCU for short) can only ever make one contribution to reaching climate targets. However, alongside the expansion of renewable energies and the increase in energy efficiency, CCU can nevertheless represent an essential step towards achieving the reduction targets in industrial companies.
Figure 3: Global greenhouse gas emissions by sector, based on the Climate Analysis Indicators Tool (CAIT). Adapted froms 5.
2. As a valuable raw material, CO2 opens up additional sources of income
The capture and subsequent utilization of CO2, or CCU, creates a wide range of economic opportunities. For example, CO2 may serve as a raw material for a range of products and services, directly or indirectly by converting CO2 into useful CO₂ products.
A primary application of CO2 is, for example, in fire extinguishing systems or greenhouses. Indirect use includes the synthesis of energy sources and basic chemicals or (intermediate) products of the chemical industry.
CO2 can either be collected by polluters, for example, in steel mills or extracted from the air using Direct Air Capture (DAC). The higher the proportion of CO2 in the air, the easier it is to filter out. However, since the CO2 content in the atmosphere is very low at 0.04%, the cost of filtering it from the ambient air is correspondingly high. DAC will therefore remain a niche from today's perspective. 6
In comparison, CO2 recovery from flue gases, where the concentration is usually more than 10 %, is much less expensive than DAC. Primary sources of CO2 are defined point sources, e.g., flue gas flows in power plants or industrial processes, where CO2 is produced as a bywaste-product.
Figure 4: This overview includes CO2 sources (A), CO2 capture (B) and examples of usage processes (C) leading to different CCU products in four categories (D), each with the substitute product in the conventional economy (E) and the service life (F). Adapted from 7.
3. CCU technologies provide solutions for carbon-based industries
In recent years, industrial sectors have developed strategies for decarbonizing their companies. In many cases, the focus is on technologies that prevent process-related CO2 emissions from occurring in the first place. As a bridging technology, CCU can accelerate decarbonization in the medium term. However, some emissions are unlikely to be avoided in individual sectors, particularly in the cement, steel, lime and glass industries.
In addition, there will also be products in the future whose production requires carbon, which is released again at the end of the product cycle. The binding time of the CO2 ultimately depends on the product. While CO2 is released after days to weeks in case of fuels, this can take decades for building materials.
Table 1: Oil refineries, cement and steel industries offer the greatest potential for CO2 recovery 8
As a technology contributing significantly to achieving climate neutrality, CCU offers a means of solving unavoidable CO2 emissions and meeting the continuing need for carbon-based products without tapping into fossil sources. Carbon becomes a renewable source through the utilization of CO2 and meets the demand for carbon-based products, such as chemicals from organic chemistry, in a way that is less harmful to the environment. CCU thus not only enables the prevention of CO2 emissions but also replaces fossil raw materials (defossilization).
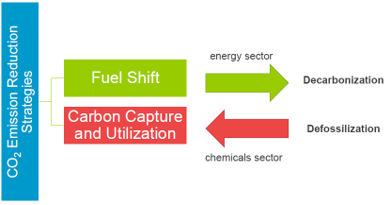
Figure 5: The carbon needed to produce chemicals and derived products must come from renewable carbon (i.e., biomass, CO2 and recycling of carbon-containing waste streams) and not from fossil sources.
Due to the drastic increase in prices for CO2 certificates, it is worth considering CCU technologies as an additional option, especially in carbon-based industries. Furthermore, the gradual shortage of freely allocated certificates results in an increase of the share of purchased CO2 certificates over time, making investments in climate-neutral technologies necessary.
After tripling last year and hitting a peak of €96.7 in February 2022, the price of traded certificates is currently around €70 per metric ton. According to estimates from Credit Suisse, the global market for CO2 emission rights could rise to +1,400% by 2030 and increase 100-fold by 2050. 9
Abbildung 6: Verlauf der CO2 Emissionsrechte 2020-2022 in Euro (Stand 9.9.2022). 10
4. CO2 products have huge market potentiall
The future market for CO2-based products and services is not easy to assess due to the early stage of technology development for many applications and the dependence on political framework conditions. Global estimates range from less than 1 Gt CO2 per year to 7 Gt CO2 per year by 2030, depending on the underlying assumptions.
In the study "CO2 Capture & Utilization: The Emergence of a Carbon Economy," for example, Lux Research, a global provider of technology-based research and consulting services, predicts the adoption of CCU technology in the fields of building materials, chemicals, carbon additives, fuels, polymers and proteins. 11
The global market for CO2 utilization will reach $70 billion by 2030 and $550 billion by 2040, according to Lux Research. As governments and industry turn their attention to technology, cement and other building materials are considered to have the greatest investment potential.
According to the study "Global Roadmap for Implementing CO2 Utilization," strategic measures to accelerate the commercialization of CO2-based products offer significantly greater market potential. For example, in the best-case scenario, five products (concrete, carbon additives, polymers, fuels, methanol) could create a market worth more than $800 billion by 2030. 12
By 2025, for example, this would mean quadrupling the market for CO2-based fuels (from $50 billion to $200 billion), simultaneously increasing CO2 reductions 15-fold (from 0.03 billion tons to 0.5 billion tons).
4.1. Potential revenues of relevant product groups
Based on the extrapolation of the studies, as mentioned earlier, the following list shows the magnitudes and ranges of potential revenues. The highest economic and environmental potential is recorded in the building materials, fuels, and chemicals sectors. For meaningful exploitation, however, the following two criteria must be met in addition to numerous other factors:
-
CO2 footprint: The CO2-based product must have a lower ecological footprint than the established products. The use of CO2 as a raw material does not automatically lead to a negative overall CO2 balance. The inclusion of CO2 separation in the CO2 life cycle assessment is therefore essential.
- Cost-effectiveness: The manufacturing and sale of the product must be competitive with the established product.
Tabelle 2: Projektion des wirtschaftlichen und ökologischen Potenzials für CCU-Produkte auf der Grundlage einer früheren Marktbewertung. Adaptiert aus .13
4.2. Market potential for renewable carbon using the example of the chemical industry
Using captured CO2 as a feedstock in the chemical industry for the synthesis of certain chemical products provides an opportunity to prevent several million tons of CO2 emissions annually and simultaneously increase the independence from fossil fuels. Therefore, using captured CO2 in this way is of growing interest to the chemical industry.
An interesting idea of future sales markets is provided by the 2050 carbon supply scenario below. Assuming a more moderate growth, Figure 7 shows a global increase in demand for the chemical industry's embedded carbon (carbon bound in the molecular structure) from 450 million tons annually to 1,000 million tons annually in 2050. 14
Mechanical and chemical recycling will become the most important future carbon sources accounting for 55%, followed by direct CO2 use at 25% and biomass at 20%. To what extent this scenario becomes reality by 2050 depends primarily on the political framework and on investments in the circular economy, CCU technologies, and the bioeconomy.
Figure 7: Under the premise of moderate growth, the scenario predicts a global carbon demand for chemicals and derived products of 1,000 Mt per year for the year 2050. Adapted from 15.
Renewable carbon sources also introduce other raw materials that promote new structures in the chemical industry. These raw materials and energy sources include CO2, hydrogen, ethanol, and especially methanol, sugar, cellulose, vegetable oils as well as waste fats and oils, and chemical recycling
Figure 8: The graph shows ways to fully meet the needs of today's large-scale chemical industry with biomass, CO2 and recycled raw materials. Adapted from 16.
4.3. The relative added value of different CO2 products
The relative added value of products from CO2 conversion is a simple measurement of profit and considers the economic aspect of the valuation. The higher the relative added value, the more attractive it is for industrial implementation. A calculation of the relative added value as part of the study "Closing the loop: captured CO2 as a feedstock in the chemical industry" came to the following conclusion: 17
- The change in CO2 prices has no significant effect on the relative added values.
- The calculated results for p-salicylic acid, oxalic acid, and dimethyl carbonate are the three highest among all 23 chemicals/fuels estimated.
- The relative added values of products such as dimethyl ether, propionic acid, formaldehyde and methanol are negative because the value of the products is lower than the cost of the reactants.
Note: The relative added value is defined as the difference between the product value and the price of the required reactants (CO2 and other reactants) divided by the product value. The calculated value serves as a theoretical economic evaluation. For the calculation of the “real” relative value creation, all production costs incurred in the process must be taken into account.
Figure 9: The graph shows the relative added value of different chemicals/fuels at different CO2 prices. A points system is used to award points between 1 and 5 in ascending order, which indicate the potential of CO2 recycling reactions, for certain value ranges. Adapted from 17.
4.4. A first assessment of CO2 conversion technologies
CO2 utilization encompasses a wide range of processes and chemical reactions. In this context, the following overview of the study "CO2 Utilization Technologies in Europe: A Short Review" provides an analysis of the techno-economic feasibility, sustainability and of the social opportunities of CO2 utilization in Europe. The evaluation of the study is based on the latest developments in CO2 use and examines CO2-based technologies with a high advancement level.
Seven indicators frequently mentioned in relevant literature were defined for the evaluation, covering technological, economic, environmental, and social aspects. The following indicators thus provide a first quick overview on the performance of the CO2 conversion technologies discussed in the study.
-
Technology status: This indicator is based on the technology readiness level (TRL) and covers the period until implementation.
-
Energy performance: This indicator assesses energy demand. It also includes energy storage potential, especially in connection with power-to-fuel technology.
-
Investment costs: This indicator refers to the capital investments, i.e., the non-consumable components for implementing CCU technology (CAPEX).
-
Operational costs: This indicator refers to the costs for the ongoing operation of the CCU technology (OPEX).
-
CO2 utilization: This indicator focuses on the mass of CO2 required to produce one kilogram of a product (given by stoichiometry).
-
Sustainability: This indicator examines the use of additional solvents, catalysts, or toxic compounds that can negatively affect the environment and the utilization of scarce natural resources. It also assesses whether it is possible to avoid fossil carbon as a feedstock for reactant production.
-
Social Acceptance: This indicator examines the general perception of CCU technology among end users. Positive public perception is an essential component for the successful commercialization of these products. However, CO2-based products such as soaps made from CO2 are still perceived as "dirty" in some cases.
Figure 10: Assessment of the technical and economic feasibility, sustainability and social opportunities of CO2 use in Europe. Adapted according to 18.
5. Launch of CCU technologies gains momentum
The growing interest in CCU technologies is reflected in the increasing number of research publications published annually and is a clear indicator of positioning towards market launch.
However, numerous projects fail to make the necessary transfer from a functioning prototype to a marketable product as the universities' willingness to research rarely exceeds level 3-4 of the Technology Readiness Level (TRL) scale. At the same time, the interest expressed by industry and investors usually only begins at TRL 6 or higher. This gap, the so-called "Valley of Death," delays the transfer process from laboratory to field and could cause the eventual demise of a technology.
Currently, many CCU technologies are under development and some already have a sufficient level of market readiness. However, bypassing the "Valley of Death" and enabling a faster transition to a marketable technology will require collaborations between academia and industry, as well as creating public awareness and political support for CCU technologies.
The growing interest of governments, industry, and investors currently provides the best conditions for developing CCU technologies into marketable technologies. In North America, the NRG COSIA Carbon XPrize supports the development of novel carbon utilization opportunities with a global competition worth $20 million. Governments in Canada, Japan, the United Kingdom, the United States and the European Commission are also providing significant R&D support for carbon utilization.
With this in mind, GIG Karasek has also initiated a project cooperating with the JKU Linz to utilize CO2 through the so-called "Dream Reaction". The first pilot plant is scheduled for industrial use by the end of 2023. 20
Table 3: Descriptions of Technology Readiness Levels (TRL) and examples of CCU technologies for each TRL. Adapted according to 7] .
6. Conclusion: CO2 as a valuable raw material for industrial processes
New options for using CO2 in the development of products and services are attracting the attention of governments, industry and investors. With CCU technologies, companies can convert millions of tons of CO2 annually into high-quality products that can be reused directly in industrial processes or used to manufacture other products. Fuels, chemicals, and carbon additives have huge potential, but innovation is required for a broad introduction of the technology. However, companies that commit themselves now will not only reduce their CO2 emissions but will also benefit from marketable products.
1 Stein, T. Carbon dioxide peaks near 420 parts per million at Mauna Loa observatory. NOAA Research. https://research.noaa.gov/article/ArtMID/587/ArticleID/2764/Coronavirus-response-barely-slows-rising-carbon-dioxide (6.9.2022)
2 Wikimedia Commons.File:Carbon Dioxide 800kyr.svg - Wikimedia Commons. https://commons.wikimedia.org/wiki/File:Carbon_Dioxide_800kyr.svg (6.9.2022).
3 Umweltbundesamt. Atmosphärische Treibhausgas-Konzentrationen. https://www.umweltbundesamt.de/daten/klima/atmosphaerische-treibhausgas-konzentrationen#methan (6.9.2022).
4 Deutsche IPCC-Koordinierungsstelle. Sonderbericht 1,5 °C globale Erwärmung – SR1.5.. https://www.de-ipcc.de/256.php (6.9.2022)
5 Our World in Data. Emissions by sector. https://ourworldindata.org/emissions-by-sector (6.9.2022)
6 Heinrich-Böll-Stiftung. CCS: Abscheidung und Speicherung von CO2 (2021). https://www.boell.de/de/2021/01/08/direct-air-capture (6.9.2022).
7 De Kleijne, K., Hanssen, S. V., van Dinteren, L., Huijbregts, M. A. J., van Zelm, R., & de Coninck, H.. Limits to Paris compatibility of CO2 capture and utilization. One Earth 2022, 5, 168–185. https://doi.org/10.1016/j.oneear.2022.01.006
8 Michele Aresta, Iftekhar Karimi, Sibudjing Kawi. An Economy Based on Carbon Dioxide and Water Potential of Large Scale Carbon Dioxide Utilization. Springer International Publishing 2019.
9 Finanznachrichten.de. Energiekrise: CO2-Steuern jagen Preise für Heizöl, Erdgas und Diesel in die Höhe. https://www.finanznachrichten.de/nachrichten-2022-03/55499071-energiekrise-co2-steuern-jagen-preise-fuer-heizoel-erdgas-und-diesel-in-die-hoehe-jetzt-profitieren-671.htm (6.9.2022)
10 boerse.de. CO2 Emissionsrechte EUR Bewertung. https://www.boerse.de/chart-tool/Co2-Emissionsrechtepreis/XC000A0C4KJ2 (6.9.2022)
11 Lux Research. CO2 capture and utilization: The emergence of a carbon economy. https://www.luxresearchinc.com/resources/chemicals/co2-capture-and-utilization-the-emergence-of-a-carbon-economy/ (6.9.2022)
12 Curty, T. Global Roadmap for Implementing CO2 Utilization. Lmc.today; Courant Constructif 2020. https://lmc.today/global-roadmap-for-implementing-co2-utilization/ (6.9.2022)
13 Spiers memorial lecture: CO2 utilization: Why, why now, and how? . RSC Publishing Home – Chemical Science Journals, Books and Databases. https://pubs.rsc.org/fa/content/articlelanding/2021/fd/d1fd00029b#cit11 (7.9.2022)
14 Vogt, D. Erneuerbarer Kohlenstoff – Schlüssel zur Zukunft (PDF). Renewable Carbon Publications. https://renewable-carbon.eu/publications/product/erneuerbarer-kohlenstoff-schlussel-zur-zukunft-pdf/ (6.9.2022)
15 Vogt, D. Global Carbon Demand for Chemicals and Derived Materials (PNG). Renewable Carbon Publications. https://renewable-carbon.eu/publications/product/global-carbon-demand-for-chemicals-and-derived-materials-png/ (7.9.2022)
16 Vogt, D. Renewable carbon refinery (PNG). Renewable Carbon Publications. https://renewable-carbon.eu/publications/product/renewable-carbon-refinery-png/ (7.9.2022)
17 Otto, A., Grube, T., Schiebahn, S., & Stolten, D. (2015). Closing the loop: captured CO2 as a feedstock in the chemical industry. Energy & Environmental Science, 8(11), 3283–3297. https://doi.org/10.1039/c5ee02591e
18 Zheng, Y., Yu B., Wang J., Zhang J. (2019). Economic Analysis of CO2 Conversion to Useful Fuels/Chemicals. researchgate.net. https://www.researchgate.net/publication/334347891_Economic_Analysis_of_CO2_Conversion_to_Useful_FuelsChemicals
19 Chauvy, R., De Weireld, G. (2020). CO2 Utilization Technologies in Europe: A Short Review. Research.net. https://www.researchgate.net/publication/344273392_CO2_Utilization_Technologies_in_Europe_A_Short_Review (7.9.2022)
20 GIG Karasek. CO2 Verwertung: Wenn aus CO2 wieder Wertstoffe werden. https://www.gigkarasek.com/de-at/blog/co2-verwertung (7.9.2022)